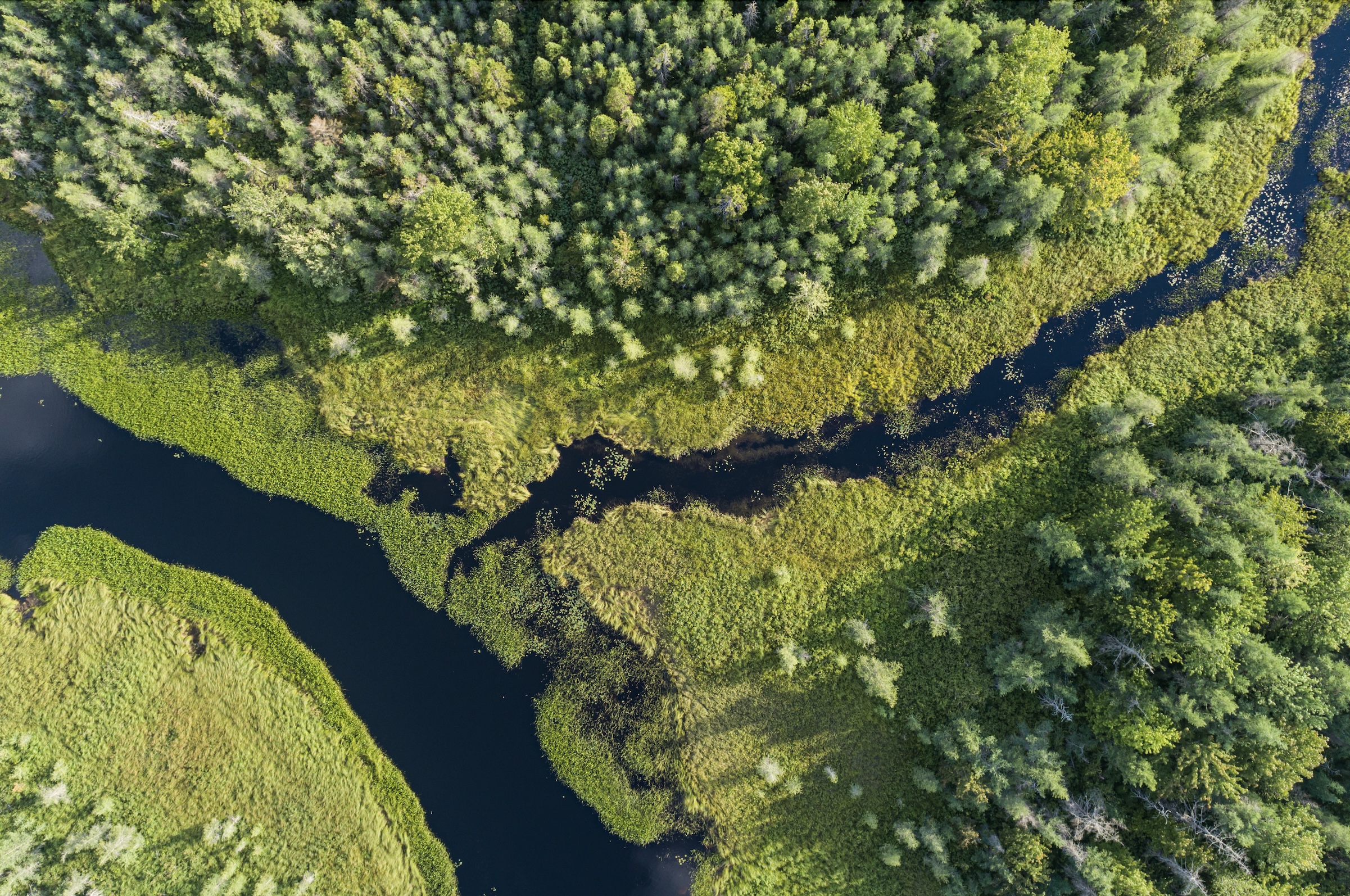
Introduction
Methane (CH4) is a significant greenhouse gas with a global warming potential over 80 times higher over a 20-year period.1 Methane has contributed over 30% of anthropogenic warming to date,2 and has potential to be one of our fastest and most effective mitigation tools to avoid the worst effects of climate change.3 Despite this, methane's role in climate change is often under appreciated. Unlike CO2, where accumulation over time is the primary concern, methane's impact is driven by the differences in rates of its production and destruction. Although methane warms the atmosphere much more than CO2 while it is present, it breaks down within 10-12 years (compared to CO2 which can last for hundreds of years).
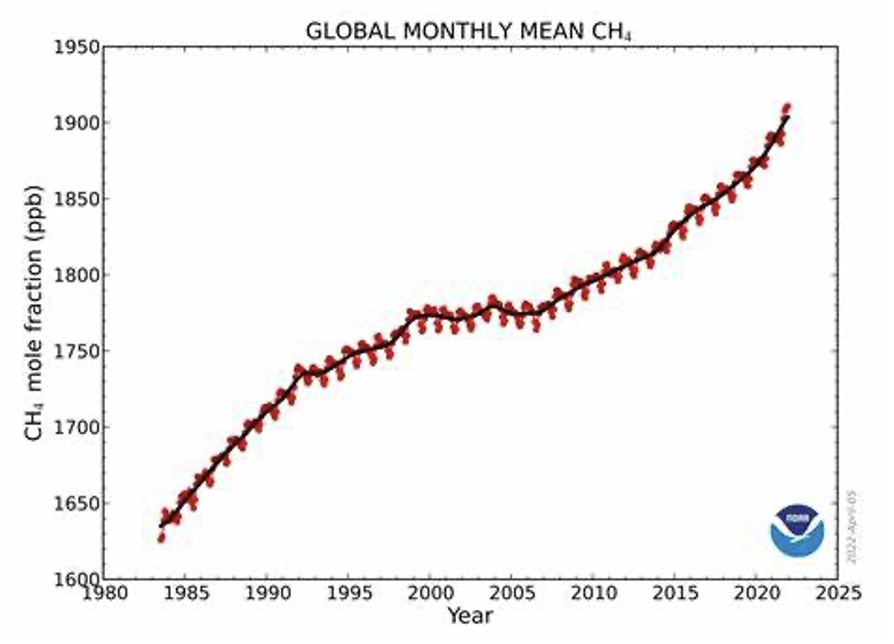
Unfortunately, the current balance of rates is biased to production which has been leading to significant and accelerating increases of methane concentrations in the atmosphere (Figure 1). While there was a temporary pause in methane growth from roughly 1998-2008, atmospheric concentrations resumed their increase and appear to be accelerating, indicating that methane production rates are growing faster than the rates of removal from the atmosphere.
Anthropogenic and Natural Sources
Methane comes from a wide range of sources including both human-caused (anthropogenic) or natural (Figure 2).
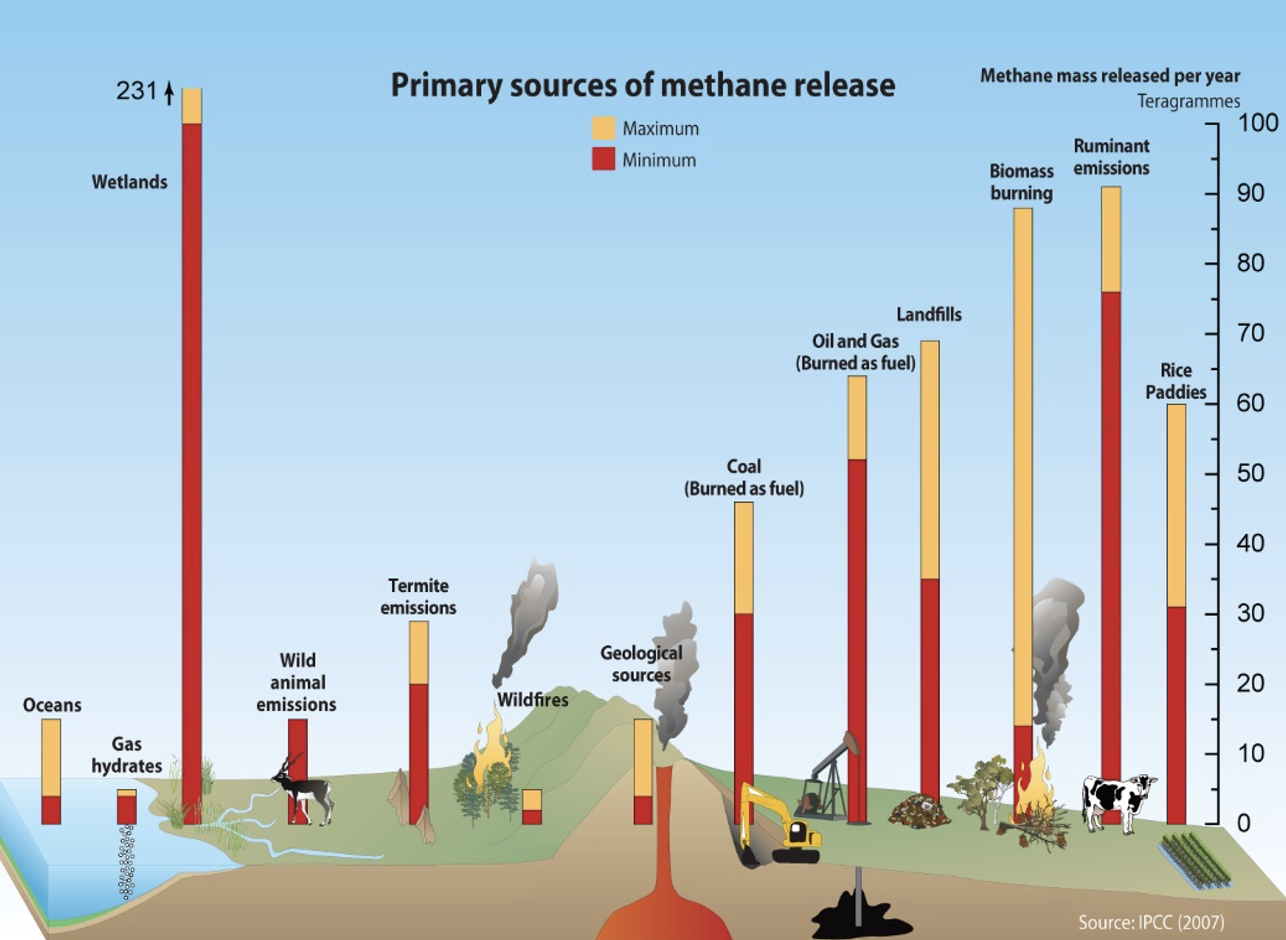
Current literature and regulatory frameworks primarily focus on anthropogenic methane sources, particularly the oil and gas industry and livestock, especially cattle. These sources are generally targeted due to their significant contribution to methane emissions, but more importantly their relative accessibility to human intervention. There is a perception that since these emissions are caused by human activities, they are likely more impactful to remove from the environment since they are ‘unnatural’. Natural methane sources, however, are potentially larger and receive much less attention. Understanding and addressing these natural sources is crucial for comprehensive methane management.
Biological Sources of Atmospheric Methane Growth
Wetlands and Methane Emissions
Wetlands, for instance, are a significant natural source of methane, making up roughly 20-30% of global methane emissions and potentially more than oil & gas and livestock combined. The growth in atmospheric methane levels can largely be attributed to increased emissions from these natural sources rather than anthropogenic activities. A recent study showed that wetland emissions increased by approximately 10 Tg in 2020 while anthropogenic emissions from all sources decreased by only 1 Tg.4 This comparison is critical as the current increases from wetland emissions are significantly larger than even the most aggressive proposed reductions in emissions from the Global Methane Pledge5 and threaten to more than offset any benefit from planned elimination of anthropogenic emissions.
The Net Balance of Methane Production and Consumption
Methane Uptake in Soils
Methane emissions from natural environments such as wetlands are the net result of methane production and consumption. Methane is produced from decaying organic matter by methanogenic bacteria in regions without oxygen, such as deep in flooded soils. In the upper regions of the soil or water table where oxygen is present, methanotrophic microorganisms (mems) consume methane, helping to regulate its atmospheric concentration. Estimates suggest more than 80% of wetland methane never reaches the atmosphere6 because mems in the soil function as an effective filter to capture methane before it is released into the atmosphere.7 Soil mems consume up to ~30 Tg atmospheric methane per year8 over and above the methane that they prevent from being emitted in the first place.
Discrepancies in Methane Uptake
Soil warming due to climate change is expected to increase both production and consumption rates of methane, since warmer temperatures tend to increase bacterial growth and activity. This would then be expected to yield no significant increase in net emissions, as increased methane production by methanogens would be matched by increased methane consumption by mems. Contrary to expectations, however, methane uptake rates are not keeping pace with production increases as evidenced by the observed increase in net wetland emissions described above. Although research is ongoing, there are multiple indications that natural mem populations have decreased dramatically,9 thereby preventing or inhibiting their ability to react to increased methane production.
Impact of Intensive Agriculture
Direct Inhibition of Mems
Intensive agriculture, particularly the use of ammonia-based fertilizers, inhibits mem activity.10 This inhibition is more pronounced in urban and agricultural areas, reducing methane uptake and contributing to higher atmospheric methane levels.11 Long-term effects were observed particularly for atmospheric methane oxidation in various upland soils.12 Another example are termites that are known to be a major source of methane but not to contain mem activity.13 It has been assumed that, among other reasons, high ammonia concentration maybe responsible for the absence of methane oxidation in termite hindguts.14 Mem activity in soil has also been shown to strongly depend on soil structure and pore size,15 both of which are negatively affected by intensive agricultural practices which lead to degraded soil quality over time.
Climate Models and the Soil Methane Sink
Oversight in Current Models
Current climate models treat the soil sink as a static number which has not changed significantly over the past 30 years.16 However, multiple sources have pointed out large discrepancies between top-down and bottom-up estimates of methane inventories,17 which may be explained if the soil sink has been dramatically reduced due to decimation of natural mem populations. In addition, current models, which assume static mem population/activity, fail to account for the surprising and accelerating net methane emissions currently observed from wetlands.
Rate Mechanism Impact
IPCC currently estimates the soil sink at approximately -40 Tg methane per year, while the observed atmospheric growth rate is estimated at +17 Tg methane per year.18 Since atmospheric concentrations of methane were constant from 1998-2008(Figure 1), the atmospheric growth rate was zero during that time and we can assume that the soil sink was an accurate approximation. However, a 50% reduction of the soil sink would directly explain the observed jump from 0 to+17 Tg methane per year. Even though the soil sink is less than 10% of the overall mechanisms for methane breakdown in the atmosphere, methane's behavior as a rate-dependent gas means that small changes in methane production or consumption can lead to substantial atmospheric impacts.
Opportunities for Intervention
Potential for Significant Impact
While the sensitivity of atmospheric levels to small changes in relative rates has resulted in significant negative impacts to date, it also provides an opportunity for rapid intervention and improvements. Relatively small interventions to increase methane uptake can lead to significant reductions in atmospheric concentrations, offering a feasible strategy for climate mitigation.
Restoring Soil Methane Sink
Restoring the soil methane sink to its former levels could effectively reduce atmospheric methane. This involves targeted efforts to promote mem populations in agricultural soils as well as natural wetlands and other locations. Importantly, this approach does not require new technologies or changes to the natural environment. Rather, the strategy should be viewed as returning the native soil mems to a healthy population level using natural resources. In the same vein as when trees are replanted following a fire or other natural disaster, it is incumbent on us as stewards of the land to attempt to restore the natural environment following the unforeseen impacts of modern agriculture.
Some implementation solutions may include:
· Reducing Ammonia and Synthetic Fertilizers
Reducing the use of ammonia-based and synthetic fertilizers can alleviate the inhibition of mems, allowing for increased methane uptake.19 Increased usage of organic fertilizers with low soluble nitrogen content can provide significant additional benefits to farmers, including higher nitrogen use efficiency; improved soil microbiomes, carbon, and water-holding capacity; and reduced nitrogen run-off and nitrous oxide formation. Importantly, reducing application of synthetic mineral fertilizers on farms should also reduce agricultural runoff, leading to a reduction in toxic effects on native mem populations in adjacent regions.
· Nutrient Enrichment
Adding essential nutrients and biobased residues to soils can selectively promote mem populations, enhancing their methane consumption capacity.20 These nutrients can be provided by organic fertilizers as discussed above, but also can be supplied as inexpensive mineral supplements that can promote selective growth of mems over methanogens for broader application to wetlands and other non-agricultural lands.
· Soil Re-seeding
Re-seeding soils with healthy mem populations is a potential strategy to restore methane uptake rates. Further research is needed to identify the most effective methods for re-populating mems, as well as identifying preferred mem strains that can tolerate conditions in current environments.
Conclusion
Methane is a critical but under appreciated factor in climate change. By understanding and addressing the complex dynamics of methane emissions, particularly the role of natural sources and soil methane sinks, we can develop effective strategies to mitigate its impact. Restoring soil methane uptake through targeted agricultural practices offers a promising pathway to reduce atmospheric methane concentrations and combat climate change. Further research and policy initiatives are needed to realize this potential.
Sources:
[1] IPCC (2021). "Climate Change 2021: The Physical ScienceBasis." Contribution of Working Group I to the Sixth Assessment Report ofthe Intergovernmental Panel on Climate Change.
[2] GlobalMethane Tracker 2023 – Analysis - IEA
[3] Ocko, I. B. et al. “Acting rapidly to deploy readily available methane mitigation measures by sector can immediately slow global warming.” Environ. Res. Lett. 16, 054042 (2021).
[4] Shushi Peng, et al. Wetland emission and atmospheric sink changes explain methane growth in 2020. Nature volume 612, pages 477–482 (2022)
[5] Homepage | GlobalMethane Pledge
[6] Le Mer, J. & Roger, P. (2001) Production, oxidation, emission, and consumption of methane by soils: a review. European Journal of SoilBiology, 37, 25–50.
[7] Jensen S. et al. Activity and abundance of methanotrophic bacteria in a northern mountainous gradient of wetlands. Environ Microbiol Rep. 2023Jun; 15(3): 206–215.
[8] Reay, D.S. , Smith, P. , Christensen, T.R. , James, R.H. &Clark, H. (2018) Methane and global environmental change. Annual Review ofEnvironment and Resources, 43, 165–192.
[9] Xiangyin Nia and Peter M. Groffman. Declines in methane uptake in forest soils. PNAS | August 21, 2018 | vol. 115 | no. 34 |8587–8590.
[10] Bodelier PL, Laanbroek HJ. 2004. Nitrogen as a regulatory factor ofmethane oxidation in soils and sediments. FEMS Microbiol Ecol 47:265–277.
[11] Zhan et al. “Urbanization can accelerate climate change by reducingCH4 uptake.” Glob Chang Biol. 2023 Jun;29(12):3489-3502.
[12] Gulledge J, Schimel JP. 1998. Low-concentration kinetics of atmospheric CH4 oxidation in soil and mechanism of NH4+ inhibition. Appl Environ Microbiol 64:4291–4298.
[13] Brauman A, Kane MD, Labat M, Breznak JA. 1992. Genesis of acetateand methane by gut bacteria of nutritionally diverse termites. Science257:1384–1387.
[14] Pester M, Tholen A, Friedrich MW, Brune A. 2007. Methane oxidationin termite hindguts: Absence of evidence and evidence of absence. Appl EnvironMicrobiol 73:2024-2028.
[15] Von Fischer, J. et al. (2009) In situ measures of methanotroph activity in upland soils: A reaction-diffusion model and field observation of water stress. J. Geo. Res. 114(G01015).
[16] IPCC (2021). "Climate Change 2021: The Physical Science Basis." Contribution of Working Group I to the Sixth Assessment Report of the Intergovernmental Panel on Climate Change.
[17] Chang, K. et al. “Observational constraints reduce model spread butnot uncertainty in global wetland methane emission estimates.” Glob Chang Biol.2023 Aug;29(15):4298-4312.
[18] Jackson et al. 2020 Environ. Res. Lett.; GlobalMethane Budget Release, July 2020
[19] Ran, Y. et al. “Warmer and drier conditions and nitrogen fertilizer application altered methanotroph abundance and methane emissions in a vegetable soil.” Environ Sci Pollut Res Int. 2017 Jan;24(3):2770-2780.
[20] Ho, A., et al. (2013). "Unexpected stimulation of soil methane uptake as emergent property of agricultural soils following bio-based residue application." Global Change Biology 19(9):2979-2989.